Tutorial – Minimize the heat load in a cryostat
In physics and materials sciences, temperature-dependent measurements play an important role. The characterization of phase transitions, thermal activities of molecules and changes in the crystal structure requires precise temperature controlling. Read further...
Further information
The most common solution for this so far have been flow and bath cryostats. These have a range of disadvantages and are therefore used less and less frequently. Flow cryostats are known for their thermal and mechanical drifts. Bath cryostats cannot cover a wider temperature range. Both systems must regularly be supplied with liquid helium. This is expensive and time-consuming and reduces the time available for experiments.
Most of the new setups are therefore used with closed-cycle helium cryostats. As these have less cooling power than liquid-helium cryostats, both the sample and surrounding hardware must be thermally anchored. When set up correctly, even a system with dozens of electrical feedthroughs has no major impact on the cooling power and the possible base temperature.
Thermal flow
Thermal flow is determined by
Q=k*A*(T2-T1)/L
- Q: Thermal flow [Watt]
- k: Thermal conductivity [Watt/Meter + Kelvin]
- A: Cross section [Meter2]
- L: Length [Meter]
- T2-T1: Temperature gradient [Kelvin]
Thermal flow in feedthroughs should be kept to a minimum.
There are three major types of heat flow: Conduction, convection and radiation. Convection does not play a large role in a closed-cycle cryostat as the sample is typically in vacuum. (An exception are cryostats using exchange gas.) Radiation has a measurable impact, which is why cryostats are equipped with radiation shields to prevent 300 K radiation radiation, coming from the outer sample chamber, from reaching the sample. Conduction is the type of heat flow which has the largest impact and is easiest to control. The following tutorial focuses on that aspect. By the choice of suitable materials and correct thermal anchoring the heat load can be reduced by several orders of magnitude.
Electrical feedthroughs
Conductor geometry
The cross-section/length ratio should be as small as possible. The cross-section size is determined by the necessary current and resistance. The rule of thumb is: as small as possible, as large as necessary. The length can be amended by coiling parts of the wire, for example by winding it around a pen and then removing the pen. Common low-temperature materials like phosphor bronze stay in shape.
Coiled phosphor bronze wire.
Material:
The ideal material has a low thermal and high electrical conductivity. Copper is not suitable, due to its very good heat conduction. At 4.2 K, phosphor bronze has less than 1 % of the thermal conductivity of copper.
Hint: Using phosphor bronze instead of copper reduces the wire heat input by more than 99%.
Thermal conductivity [W/m*K]
Material | 300K | 30K | 4K |
---|---|---|---|
Copper (C110) | 400 | 1000 | 300 |
Phosphor bronze | 48 | 12 | 1.6 |
Manganin | 22 | 6 | 0.5 |
One disadvantage is the higher electrical resistance which can lead to wire heating and a considerable drop in voltage at higher currents. Most applications require low currents (e. g. piezo positioners and thermometers) so that the wire’s resistance is not important.
Material | AWG | Resistance [Ohm/m] 300 K | Resistance [Ohm/m] 4.2 K |
---|---|---|---|
Copper (C110) | 30 | 0.32 | 0.003 |
Copper (C110) | 34 | 0.81 | 0.0076 |
Phosphor bronze | 32 | 4 | 3.3 |
Phosphor bronze | 36 | 10 | 8.6 |
Manganin | 30 | 9.7 | 8.6 |
Manganin | 36 | 39 | 35 |
Thermal anchoring
Cold heads normally consist of two cooling levels connected in series. The first cooling level has great cooling powers but only reaches temperatures around 30 K. The second cooling level can cool down to 2.6 K, but its cooling powers are limited. To reduce thermal load on the second level, the feedthroughs can be thermally anchored on the first level. Due to the smaller temperature gradient, the thermal flow decreases until it reaches the second level/the sample that is cooled by the second level.
To correctly calculate the thermal load, the temperature-dependent conductivity must be integrated over the length of the wire. When this calculation is simplified and a resistance that linearly falls with the temperature is assumed, less than 1% of the thermal energy of a 20 cm long phosphor bronze wire comes from the second cooling level.
Material | AWG | Diam. [mm] | Therm. Load | Therm. Load |
---|---|---|---|---|
Copper (C110) | 34 | 0.16 | 26.7 | 3.5 |
Phosphor Bronze | 36 | 0.127 | 1.03 | 0.02 |
Manganin | 36 | 0.127 | 0.48 | 0.01 |
The total resistance of the 20 cm long PB wire with 0.127 mm diameter (0.05”) is 2 Ohm. The thermal load is a mere 20 µW.
For the best result, the wire is anchored again to the 3K-sample platform before it is lead to the sample. Depending on the thermal conductivity and thickness of the sample, there often is a small temperature gradient between the sample surface and the backside where it touches the sample holder. This happens often when there is an extra heat input, for example by laser light excitation or microwaves. In these cases, the sample surface is slightly cooled by the 3K-anchored wire.
If a thermal clamp is not available, or if there is no space for it, wires can also be glued to the cold parts with a few drops of GE Varnish. Cold shields or sample holders are particularly suited for this. GE Varnish has a good thermal conductivity, no magnetic moment and does not become brittle at low temperatures. It can be completely removed with solvents like acetone or isopropyl.
Thermometer wire - thermally anchored with GE Varnish. (Reddish mass on top right and left on sample holder)
Correctly installed, the thermal load of the wiring can be ignored for most experiments. This is also true where a large number of feedthroughs is required.
Twisted pairs
We recommend grouping the wires in twisted pairs or buying them accordingly. Twisted pairs provide a certain shielding against magnetic fields and as such provide low noise. They are also easier to handle thanks to a limited number of cable bundles.
Contact
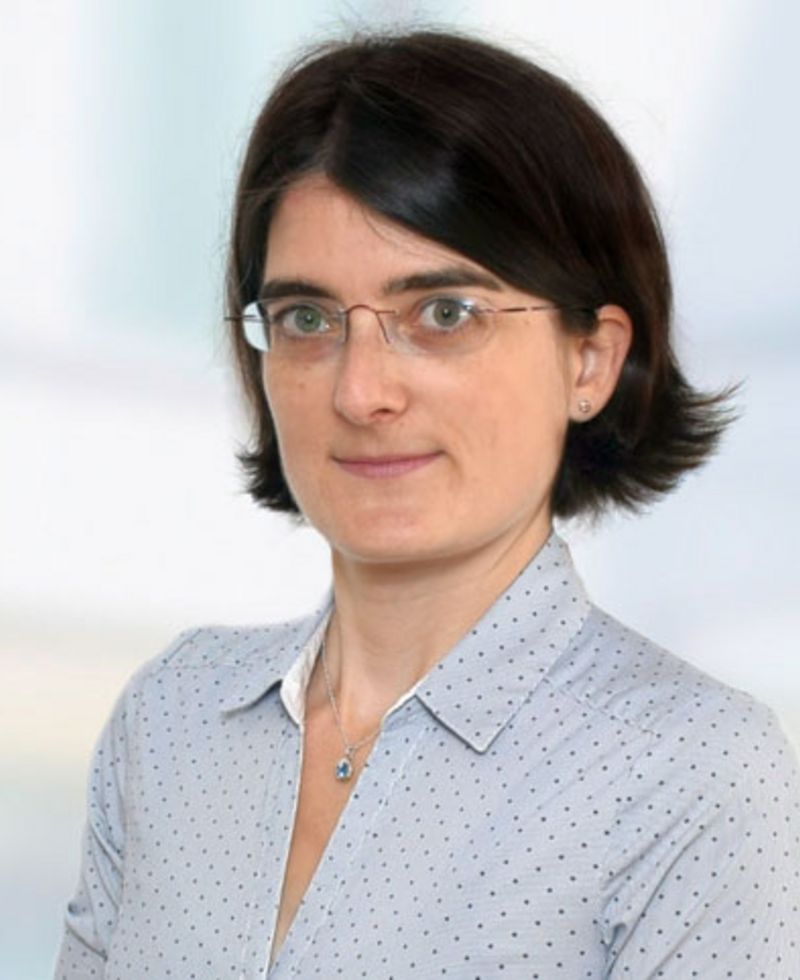
Navigation
Categories
Contact
Quantum Design GmbH
Eszak u. 24
H-1038 Budapest
Hungary
Phone: | +36 1 2402711 |
Fax: | +36 1 2402711 |
E-mail: | hungary@qd-europe.com |